Observations throughout most of the infrared wavelength range are impossible from ground-based observatories due to absorption by water vapor in the troposphere. Although SOFIA usually flew above the moist troposphere, a measurable amount of water vapor was present in the stratosphere at densities of approximately 2 to 10 parts per million. This translates into a typical precipitable water vapor level of about 7 microns. This residual water vapor overburden was sufficient to have a noticeable effect on most far-infrared observations (e.g. broadband photometry or spectroscopy of lines blended with strong water vapor absorption lines). The effects were estimated by running ATRAN to compute the atmospheric throughput over the wavelength range of interest.
Variations in Overburden
The water vapor burden at altitude for use in the calibration was affected by the season, latitude, the jet stream, and somewhat by local weather conditions at lower altitudes. In general, the tropopause is higher in the local summer, and higher water vapor burdens could be encountered. This was also true in the tropics throughout the year. In temperate latitudes, the zenith water vapor overburden at observing altitudes is typically 5 to 15 µm, but may have exceeded 20 µm if the aircraft was not above the tropopause. At 41,000 feet or higher, the WV overburden occasionally dropped below 5 µm. The distribution of stratospheric water vapor can be quite variable, and the observed zenith burden may change as much as a factor of three on time scales as short as 15 minutes. During flight, the current observed water vapor value appeared in the housekeeping video display, updated every 15 seconds. These measurements were also available after each flight, as plots from the housekeeping data file for the flight.
Radiometer Design
Atmospheric water vapor measurements were obtained with an infrared radiometry system developed by Dr. Thomas Roellig (NASA/ARC). The sensor was a heterodyne mixer configured for the measurement of the 183 GHz rotational line of water. The Water Vapor Monitor (WVM) was mounted at a fixed elevation of 40° in the upper deck of the aircraft and was responsible for measuring the integrated water vapor while the SOFIA aircraft was at normal operational altitudes. These data were used to correct the astronomical infrared data obtained by the telescope and were also used in the algorithm that determined successful SOFIA observatory flight hours for contractual purposes. The WVM reported its measured water vapor overburden to the aircraft Mission Controls and Communication System (MCCS) once every 15 seconds while the SOFIA observatory was in normal operation at altitude.
Principle of Operations
The WVM determined water vapor overburden by comparing radiometric measurements of the center and wings of the 183.3 GHz rotational line of water observed in the atmosphere to atmospheric models.
Since in practice the aircraft was not flying perfectly level all the time, the WVM measurements had to be corrected for the true aircraft roll and pitch angles during the measurements. The aircraft roll and pitch angles were provided to the WVM by the aircraft flight system autopilot, which could measure these parameters to better than 0.1°.
The Radiometer Head contained an antenna that viewed the sky, two calibrated reference targets (one heated and one ambient temperature microwave blackbody), an RF switch, a mixer, a local oscillator, an IF amplifier, and an inclinometer. All of these components were mounted together on a baseplate and were attached to the inner surface of the aircraft fuselage, so that the antenna could observe the sky through a microwave-transparent window. The antenna itself employed a quasi-optical design with a microwave lens that fed a feed horn. The beam pattern was Gaussian with a full-width-half-maximum diameter of 0.87°. A sub-harmonic mixer mounted directly behind the feed horn mixed the 183.3 GHz radiation down to a bandwidth of 1 GHz with the radiometer operating in double sideband mode. The sub-harmonic mixer was fed by a phase-locked 91.65 GHz local oscillator. The intermediate frequency signal out of the mixer was amplified by an RF amplifier with a bandwidth of 100kHz - 500MHz before it was sent on to the IF Converter Box.
Calibration
Since the WVM operated as a radiometer, accurate gain stability was important. In order to achieve this stability the two reference blackbodies were used to periodically insert a stable signal into the radiometer. Small motors rotated mirrors that directed images of the black body targets into the feedhorn field-of-view once every 5 seconds. One mirror, the Sky/Calibrator Mirror directed the view of the radiometer between the sky and the black body calibrators. The second mirror, the Hot/Ambient Mirror, selected between the hot and ambient temperature black bodies. Therefore, over a 15 second period the radiometer viewed the sky for 4 seconds, an ambient temperature black body target for 4 seconds, and a heated black body target for 4 seconds. One second was allowed to move the mirrors at each viewing position. The temperatures of the two black body targets were measured with temperature sensors.
Typical Post-flight Results
A typical zenith water vapor plot for a KAO flight is shown in figure below. The plot is usually provided with temperature and altitude data as well.
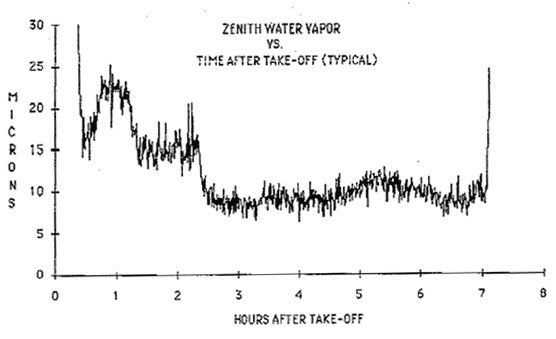