NASA’s Stratospheric Observatory for Infrared Astronomy (SOFIA) deploys to Christchurch, New Zealand, during the southern hemisphere’s winter months to take advantage of the long nights and especially cold, dry conditions in the stratosphere. Flying from Christchurch enables the observatory to study celestial objects not visible from SOFIA’s northern hemisphere base.
During deployment campaigns, SOFIA has used the German REceiver for Astronomy at Terahertz Frequencies (GREAT) high-resolution spectrometer, the Field Imaging Far-Infrared Line Spectrometer (FIFI-LS) , and the Faint Object InfraRed CAmera for the SOFIA Telescope (FORCAST) employed in both imaging and spectroscopic modes.
The following scientific results were obtained during SOFIA flights operating from Christchurch. These observations studied star formation, energy flow and chemistry in the interstellar medium, late stages of stellar evolution, and supernovae.
STAR FORMATION
Astronomers have a good general understanding of how stars form. Evidence gathered over decades shows that clouds of gas and dust in space contract because of their own gravity. Individual clumps condense inside those collapsing clouds and become stars. Nevertheless, despite decades of careful study, there are many details of the star formation process that are not well-understood. Following are some examples of open questions about star formation.
Star formation rate: Do interstellar medium clouds that become protostars rapidly collapse in “free-fall”, or is the collapse significantly slowed by other factors? To answer that question, astronomers need celestial "clocks" that will let them determine the ages of star-forming clouds. One such clock is provided by the molecule H2D+, a combination of two ordinary "light" hydrogen atoms symbolized by H, plus one "heavy" hydrogen atom, symbolized by D for deuterium. The spin axes of the two H atom nuclei within each molecule can flip their relative orientations. The proportion of H2D+ molecules with one spin orientation (called “para”) versus the other orientation (called “ortho”) changes at a known rate, giving the age of the cloud containing those molecules. Because water vapor in Earth’s atmosphere absorbs all far-infrared radiation from celestial sources, the only observatory able to detect the H2D+ para line needed to complete the clock measurement is SOFIA, flying in the dry stratosphere.
Cooling lines: Estimates based on the number, sizes, and masses of interstellar clouds indicate that stars should be forming in the Milky Way Galaxy at about 10x the rate at which they actually are forming. Those theoretical over-estimates for the local star formation rate include assuming substantial effects of “cooling lines,” certain far-infrared and radio spectral lines that can drain energy from the center of a dense star-forming cloud. Such an energy drain would promote cloud collapse and star formation, like pulling the plug in a sink causes the water to collapse toward the center of the bowl. Astronomers speculate that the surprisingly low observed rate of star formation could partly be due to cooling lines being less effective than expected.
Protostars and bipolar jets: Stars that are forming, called protostars, sometimes emit strong “jets” of material into the surrounding interstellar medium. These high-velocity outflows usually travel from the protostar in two opposite directions, and so are called “bipolar” jets. The significance of bipolar jets from protostars is that they can compress neighboring ISM clouds with which they collide, triggering more star formation. Or, if they are energetic enough, they might instead disperse clouds they encounter and thereby actually prevent more star formation. Which of those two scenarios happens is probably highly dependent on the jet characteristics as well as details of the arrangement of the surrounding material.
The Magellanic Clouds: The Large and Small Magellanic Clouds (LMC and SMC) are satellite galaxies orbiting our Milky Way Galaxy. Star formation in the Magellanic Clouds is especially interesting to astronomers for comparison with star formation in our Galaxy because the material in the Clouds has lower metal content than in the Milky Way. The Clouds are chemically more like the primordial material from which the first generation of stars formed after the Big Bang. Astronomers want to understand the extent to which that composition difference causes star formation to operate differently in the Clouds versus within our Galaxy.
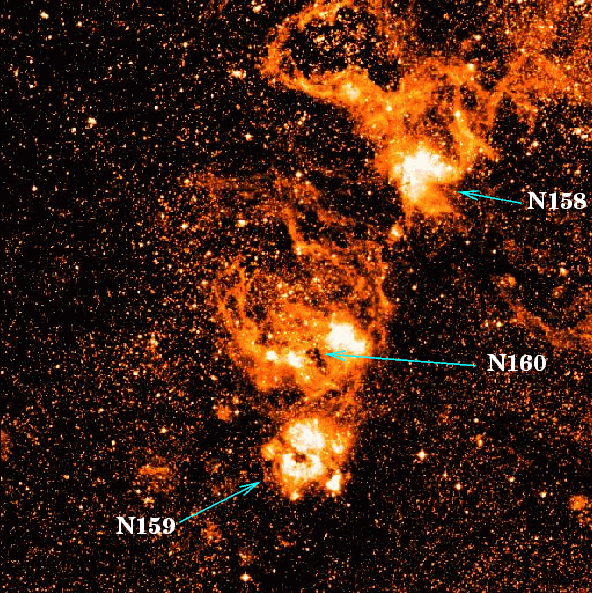
Digitized Sky Survey red visual-wavelength image of the N158, N159, and N160 star-forming regions in the Large Magellanic Cloud. Only N159 and N160 were targets of this study.
Massive Star Formation in the LMC. I. N159 and N160 Complexes
Gordon, M. S. et al. 2017, ApJ 834, 122
Gordon et al. studied 14 massive YSOs (Young Stellar Objects, i.e., late-stage protostars) in three star-forming complexes in the Large Magellanic Cloud (LMC). SOFIA/FORCAST images were used in combination with Spitzer, Herschel, and 2MASS data to determine spectral energy distributions (SEDs) of the young stars. SOFIA filled in important middle segments of those SEDs, allowing better estimates of luminosities & ages of those stars.
The ages determined for the YSOs in this study ranged from 30,000 to 700,000 years, extremely young in the context of star formation. A search was made for even younger, cooler protostars but none were found. (One star was diagnosed from these data as being an evolved red giant star rather than a young star.) Each cluster of protostars has just one high-luminosity member that dominates the near- and mid-infrared SEDs, similar to the situation in Milky Way Galaxy star-forming regions. This means that interstellar clouds collapse and fragment into individual protostars in the same way in the low-metallicity LMC as in the high-metallicity Milky Way, despite the composition difference. That means that the first generations of stars after the Big Bang, which formed from low-metallicity material, probably formed in much the same fashion that stars form in the Milky Way Galaxy today. (Note that the paper is numbered “I”, implying that the authors are writing up a series of similar studies.)
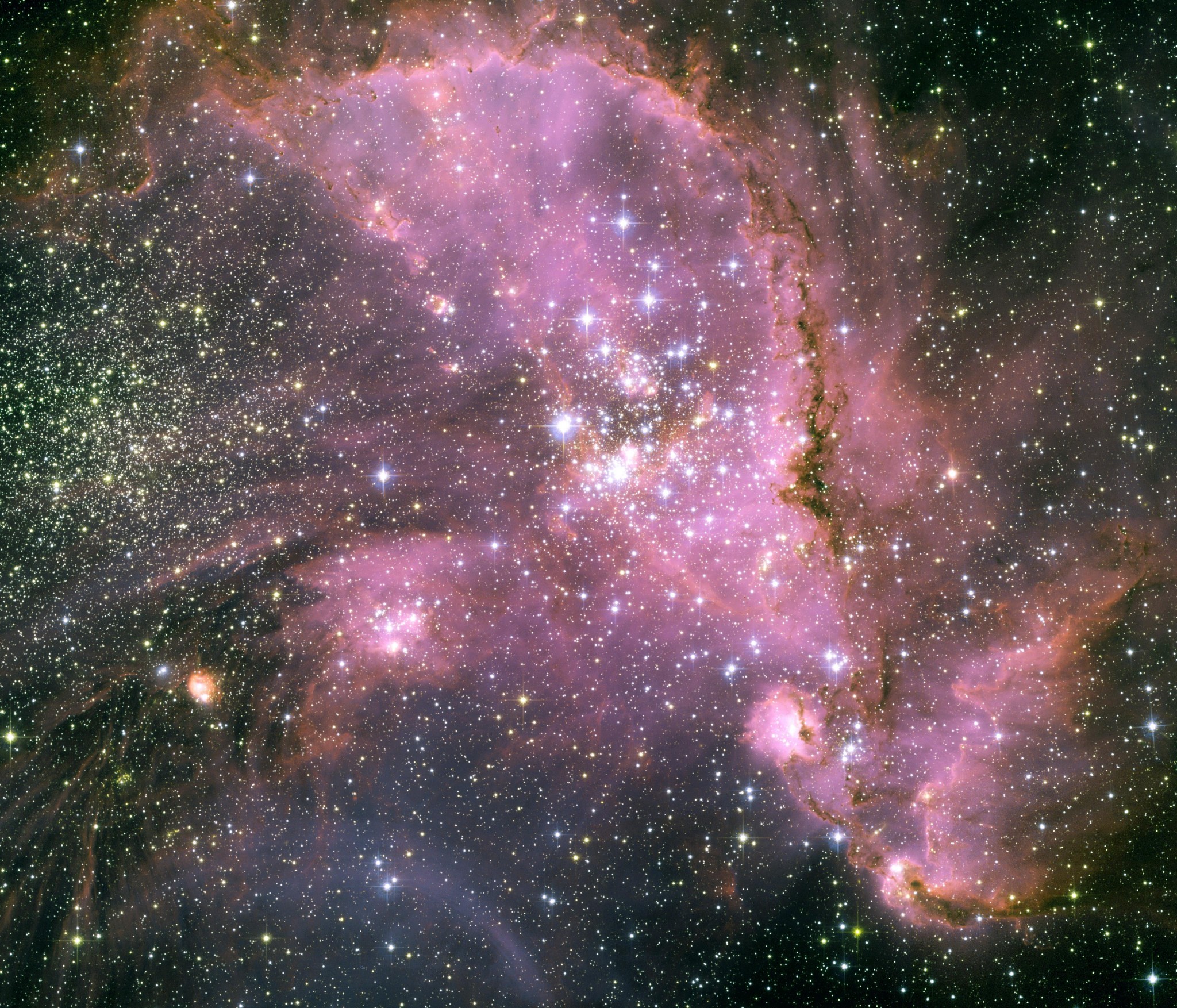
Hubble Space Telescope visual-wavelength image of star-forming region N66 in the Small Magellanic Cloud.
Carbon gas in SMC low-metallicity star-forming regions
Requena-Torres et al., 2016, A&A, 589, A28
Requena-Torres et al. studied the gas in three star-forming complexes in the Small Magellanic Cloud (SMC) named N66, N25+N26, and N88. They used SOFIA/GREAT spectra combined with observations from the APEX radio telescope in Chile to investigate the best way to measure the total mass of raw material available for new stars in these regions. This study involved making maps and spectra of neutral [C I] and ionized [C II] carbon gas with SOFIA plus maps and spectra of carbon monoxide (CO) gas with APEX. The SOFIA and APEX data were analyzed using a computer model of how radiation from newborn stars would interact with surrounding gas.
The authors concluded that, in the low-metallicity gas found in the Magellanic Clouds, ionized carbon [C II] is the best proxy, or “tracer”, for where undetectable but dominant hydrogen is located. This is unlike the Milky Way Galaxy’s higher-metallicity gas, for which carbon monoxide (CO) is the best tracer of hydrogen.
These results can be used by other astronomers to help do more accurate “bookkeeping” of how much raw material for star formation is in the Magellanic Clouds, as well as in distant galaxies of similar composition that were forming stars soon after the Big Bang.
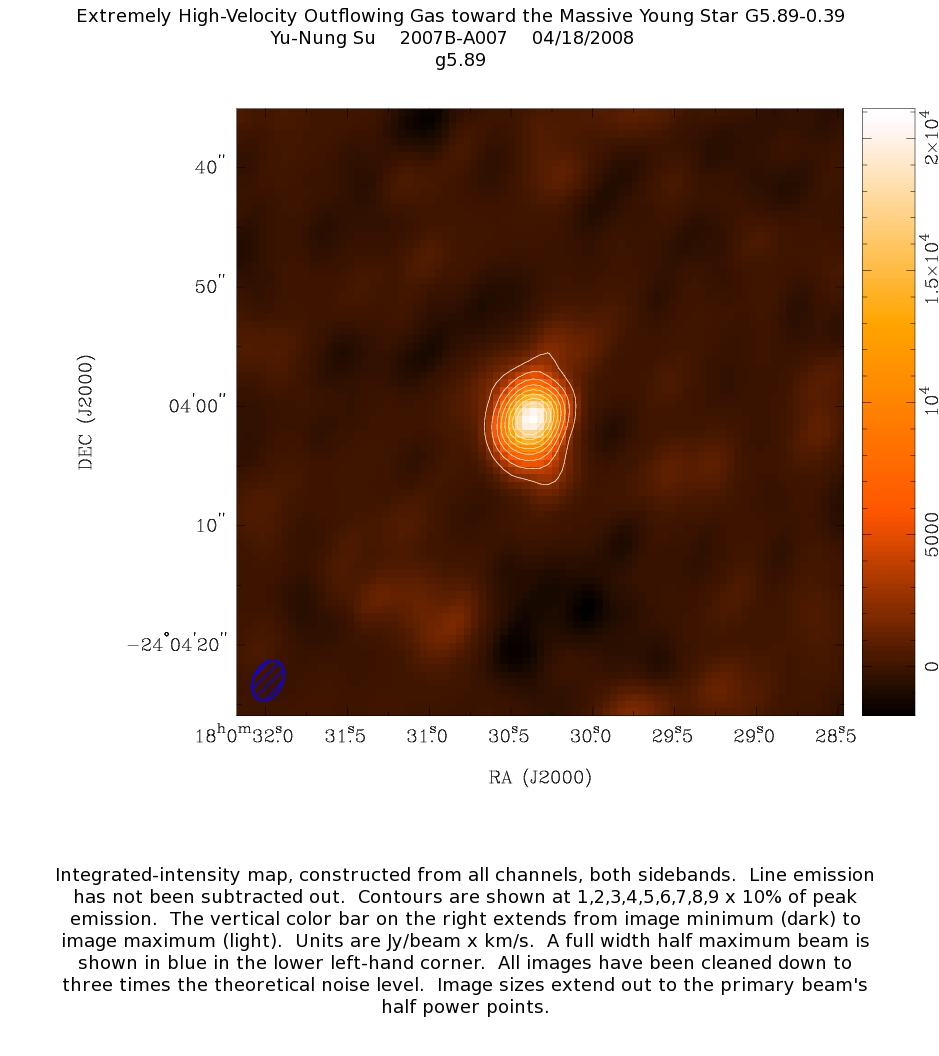
Radio-wavelength image of massive protostar G5.89-0.39.
Spectroscopically resolved far-IR observations of the massive star-forming region G5.89-0.39
Leurini, S., et al. 2015, A&A, 584, A70
Leurini et al. used the GREAT far-infrared spectrometer on SOFIA to measure the strength of a neutral atomic oxygen [O I] cooling line coming from various parts of the massive star-forming region G5.89-0.39, or G5.89 (Gee-five-point-eighty-nine) for short. To study composition variations and energy flow within G5.89, the they combined SOFIA/GREAT data with APEX radio telescope observations of carbon monoxide (CO) and Herschel infrared space telescope observations of ionized carbon (C II), water (H2O), and hydrogen fluoride (HF). Tthe GREAT instrument’s wavelength range makes it the only available instrument for detecting the [O I] line; its high spectral resolution makes it uniquely able to distinguish separate sub-clouds within G5.89 that have slightly different velocities relative to Earth.
Leurini et al. conclude that [O I] is the dominant cooling line throughout G5.89, followed by CO; other lines are relatively minor in effect. The total amount of cooling-line emission is consistent with the observed (low) rate of star formation. Extension of this study will require SOFIA and APEX observations of more star-forming regions. This result contributes to ongoing investigations by many astronomers seeking to determine why the observed rate of star formation is much lower than the rate predicted by simple calculations based on the amount of available raw material.
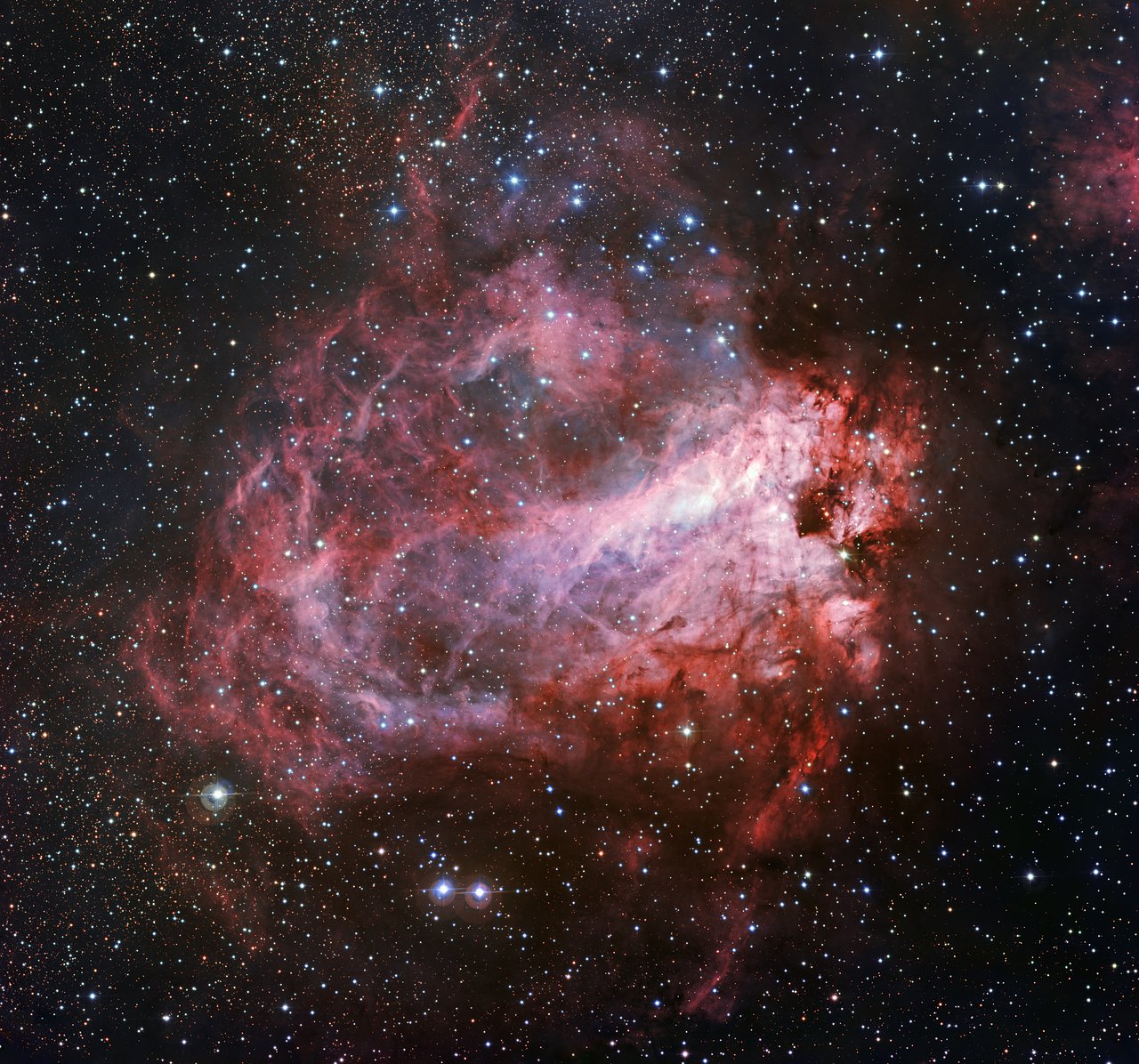
Visual-wavelength image of the Messier 17 (M17) star-forming region.
Disentangling the excitation conditions of the dense gas in M17 SW
Pérez-Beaupuits, J. P., et al., 2015, A&A, 583, A107
Pérez-Beaupuits et al. used SOFIA and the GREAT far-infrared spectrometer to study four positions in the star-forming cloud Messier 17 Southwest (M17 SW). Their goal was to compare the strengths of effects that tend to make cloudlets contract and form stars, versus effects that tend to make them expand and disperse. The investigators calculated models of the chemical and energy conditions in M17 SW based on their measurements from SOFIA/GREAT of carbon monoxide (CO) spectral lines combined with measurements from the APEX (Chile) and IRAM (Spain) radio telescopes of other CO spectral lines plus spectral lines of HCN and HCO+ molecules.
Pérez-Beaupuits found to their surprise that all of the cloudlets they examined were ‘super-virial’, meaning leaning in the direction of expanding and dispersing rather than contracting to make stars. In warm cloudlets this imbalance is apparently due primarily to heating by radiation from nearby young stars and protostars, in cold cloudlets this imbalance is due mostly to magnetic fields inside the clouds. This result contributes to ongoing investigations by many astronomers seeking to determine why the observed rate of star formation is much lower than the rate predicted by simple calculations based on the amount of available raw material. In M17 SW, impediments to continued star formation appear to include heating by new stars plus the effects of magnetic fields embedded in the raw material.
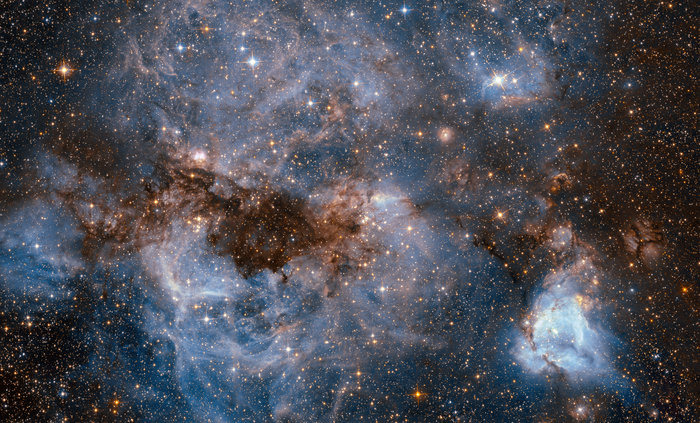
Composite near-infrared and visual-wavelength image of the N159 star-forming region in the Large Magellanic Cloud from the Hubble Space Telescope, spanning a region 150 light-years across.
Velocity resolved [C II], [C I], and CO observations of the N159 star-forming region in the Large Magellanic Cloud: A complex velocity structure and variation of the column densities
Okada, Y., et al., 2015, A&A, 580, A54
Okada et al. studied the strengths and locations of emission of cooling lines from ionized carbon [C II] and ionized nitrogen [N II] in the N159 star-forming region within the Large Magellanic Cloud (LMC) using measurements from SOFIA with the GREAT far-infrared spectrometer. They combined those data with carbon monoxide (CO) and neutral carbon [C I] mesurements from the APEX radio telescope. Essential to this study were the high spatial resolution and spectral resolution capabilities of both SOFIA/GREAT and APEX that allowed adjacent cloudlets to be distinguised.
Okada et al.’s main result is that in N159 the [C II] gas has a much wider velocity range than CO and [C I], so the [C II] can be inferred to represents more of the underlying total cloud mass (mostly hydrogen). This result can be confirmed by similar measurements of other star-forming regions in the Magellanic Clouds. If confirmed, it will provide a more accurate method for making an census of star-forming raw material in low-metallicity systems such as primordial galaxies viewed at great look-back times, soon after the Big Bang.
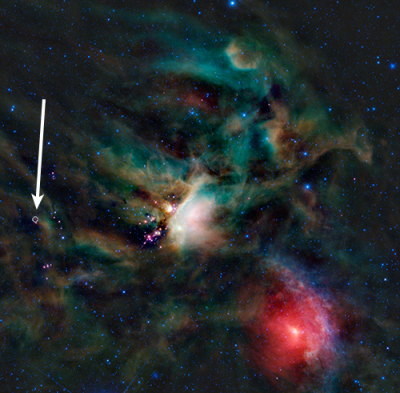
WISE space telescope near-infrared image of the Rho Ophiuchi star-forming region. Brünken et al. made measurements along the line-of-sight to protostar IRAS 16293-2422 (arrow) establishing a molecular ‘clock’ to determine the age of the protostar.
H
2
D
+
observations give an age of at least one million years for a cloud core forming Sun-like stars
Brünken, S., et al., 2014, Nature, 516, 219
Brünken et al. used the GREAT spectrometer on SOFIA to measure the amount of para-H2D+ in the star-forming cloud named IRAS 16293-2422, located at a distance of about 400 light years in the direction of the constellation Ophiuchus. (The name means this object was discovered by the Infrared Astronomy Satellite, IRAS, in 1983; the numbers give its celestial longitude and latitude.) The investigators combined their SOFIA data with measurements of ortho-H2D+ from the APEX radio telescope located in the Chilean Andes.
Brünken et al.’s observations yielded the surprising result that, although IRAS 16293-2422 is known to be forming protostars with the mass of our Sun, its age is at least 1 million years. That result conflicts with current models which predict solar-mass star formation should proceed much more rapidly, on a free-fall time scale. The implication is that the rate of star formation in that cloud is substantially slowed down by some unknown effect or combination of effects. Brünken et al. have pioneered a new, general technique for determining the ages of star-forming interstellar clouds that can be used to test models of the star-formation process. This technique requires the unique far-infrared capabilities of SOFIA and the GREAT instrument. [This significant result was published in Nature, Europe’s and the U.K.’s most prestigious science journal.]
STELLAR EVOLUTION AND SUPERNOVAE
Wolf-Rayet stars: Wolf-Rayet (WR) stars are massive, luminous stars that are rare but have an outsized effect on the heating and composition of the gas and dust in the interstellar medium from which future generations of stars will form. A WR star expels a large fraction of its own mass into space before a violent death in a supernova explosion. However, models of WR star evolution often do not match their observed properties.
Supernovae: When a massive star runs out of fuel, the subsequent collapse of its core and resulting violent supernova explosion produces what is called a supernova remnant, a cloud of hot material blasting away from the explosion site at tens of thousands of kilometers per second. Astronomers hypothesize that a rapidly expanding supernova remnant cloud can sometimes act like a snowplow, compressing material in neighboring clouds and triggering the formation of new stars.
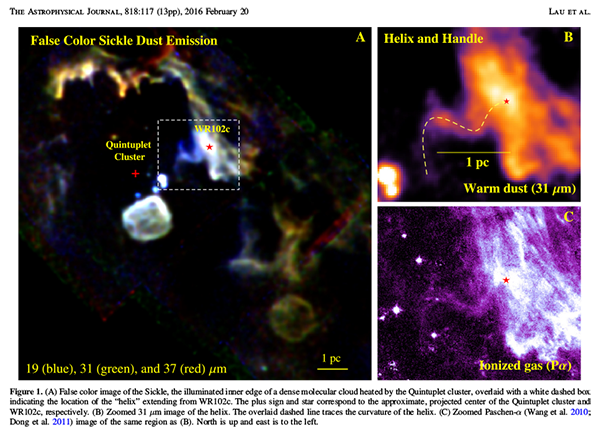
(a) False-color mid-infrared image of the region around Wolf-Rayet star WR102c near the Galactic Center, from SOFIA/FORCAST. WR102c itself is near the center of the dotted-line box. (b) Zoomed image of the WR102c box in panel (a) at a wavelength of 31 microns from SOFIA/FORCAST. (c) Near-infrared hydrogen emission-line image of the same field of view as panel (b) from Wang et al. 2010. (Figure 1 from Lau et al. 2016)
An Apparent Precessing Helical Outflow from a Massive Evolved Star: Evidence for Binary Interaction
Lau. R. M. et al., 2016, ApJ, 818, 117
Lau et al. studied a single Wolf-Rayet (WR) star near the center of the Milky Way Galaxy named WR102c. Using SOFIA/FORCAST images, they found that WR102c is shooting gas & dust in a helix (conical spiral) rather than uniformly in all directions. The authors conclude that the shape of WR102c’s outflow implies that the star is actually a binary, and the gravity of its unseen companion causes it to precess, i.e. sweep its rotation axis around in a circle like a spinning top, with a period between 800 and 1400 days. That precession motion causes the outflowing gas and dust to have a spiral shape, like the water coming out of a twirling garden hose.
The authors looked for evidence of helical outflows around other WR stars and found two candidates. They speculate that undetected binary companions may explain discrepancies between observations and models of other WR stars. This result improves our understanding of the evolution of massive WR stars that have an outsized influence on the composition and temperature of the interstellar medium, factors which control how and when new stars form. This research can be extended by infrared imaging of outflows from more WR stars.
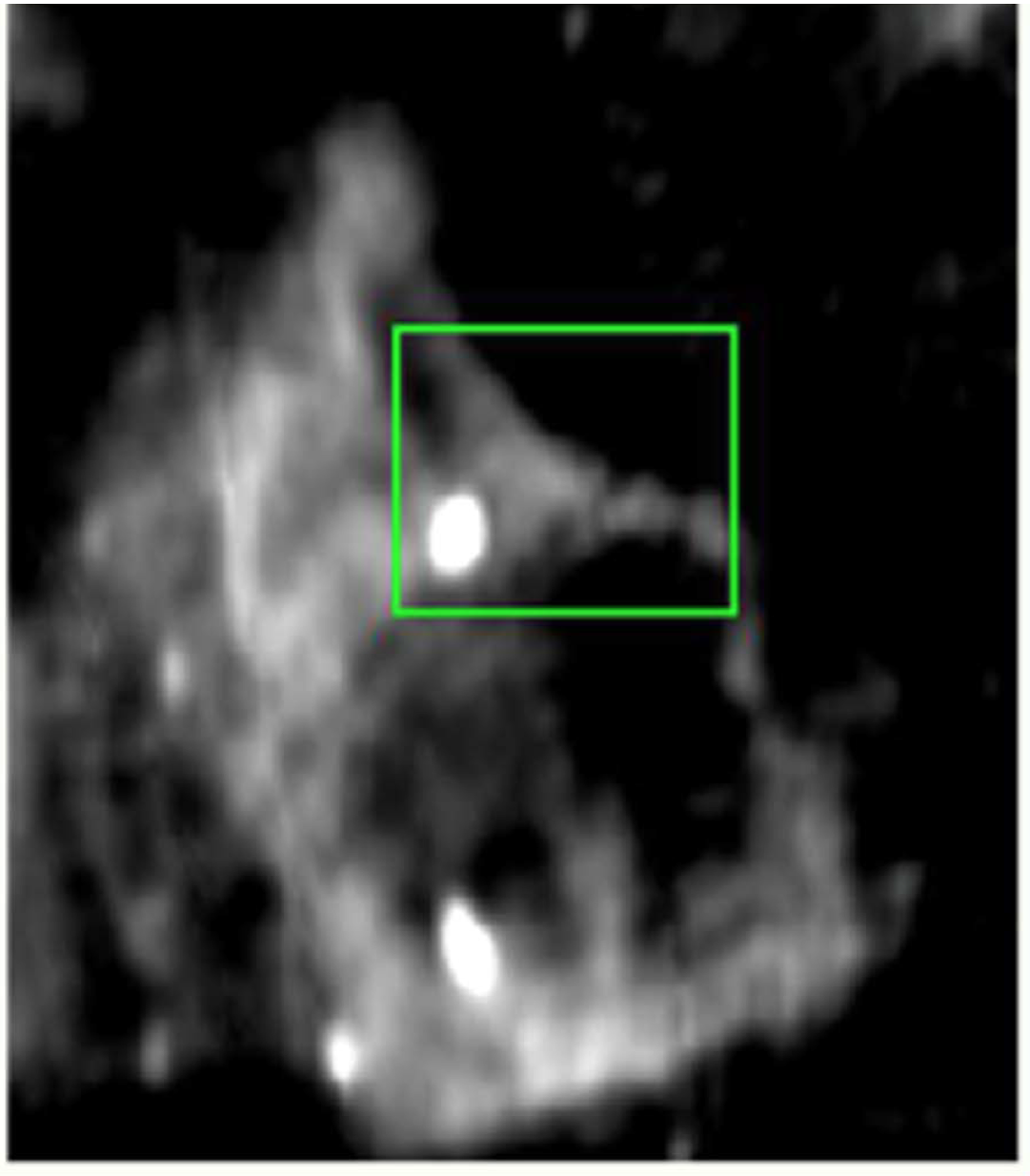
Radio-wavelength image of supernova remnant G357.7+0.3. The green box indicates the region of the SOFIA/GREAT observations. (Figure 1b from Rho et al. 2017).
Discovery of Broad Molecular Lines and of Shocked Molecular Hydrogen from the Supernova Remnant G357.7+0.3: HHSMT, APEX, Spitzer, and SOFIA Observations
Rho, J. et al. 2017, ApJ 834, 12
Rho et al. investigated the collision between the expanding cloud of gas produced by a particlar supernova explosion and surrounding interstellar material. This study involved combining SOFIA observations using the GREAT far-infrared spectrometer with data from the Spitzer infrared space telescope plus the HHSMT, APEX, and NRAO radio telescopes to understand how supernova remnant G357.7+0.3, or G357.7 for short (pronounced Gee-three-fifty-seven-point-seven) that is located near the center of our Milky Way Galaxy is affecting neighboring material.
The authors conclude that the expansion of supernova remnant G357.7 is clearly impacting some interstellar clouds that are repositories of stellar construction material. This means that the death of one massive star could eventually cause the birth of stars in neighboring clouds.
INTERSTELLAR MEDIUM (ISM) AND ASTROCHEMISTRY
The gas and dust between the stars, called the interstellar medium, is mostly invisible to human eyes. Its densest clouds are the nurseries where new stars are born. Almost all of the ISM is in the form of gas. More than 150 molecules including hydrogen (H2), carbon monoxide (CO), hydroxyl (OH), water (H2O), and even ethyl alcohol (C2H6O) have been detected in ISM clouds. However, many details of the interstellar chemistry processes by which these molecules form are not well understood. Astronomers have made mathematical models to estimate the rates at which atoms combine to form molecules in dense ISM clouds, but the models need to be checked by comparison with observations.
Water: Water is of special interest to astronomers because it is crucial for the existence of life on Earth. Models of the formation of the Solar System indicate that most of the water on Earth could have formed in the ISM cloud that gave birth to the Sun and the planets of our Solar System, long before Earth itself formed.
Organics: Abundances of sulfur-bearing molecules in the ISM have special significance because they can serve as catalysts for the formation of organic molecules, which are interesting in connection to the origin of life on Earth and possibly other planets. Astronomers hypothesize that some of the organic molecules on the surface of the young Earth might have formed in the ISM before Earth formed.
ISM cloud masses: Most of the mass of the Universe, including the material from which stars form, is hydrogen. In dense clouds such as those in star-forming regions, hydrogen is in the form of H2 molecules. Unfortunately, H2 molecules, although abundant, are difficult to detect because they have very few spectral “fingerprints.” Astronomers instead measure the amount of proxies, such as neutral carbon [C I], ionized carbon [C II], and carbon monoxide (CO), that are more easily detected than H2 in order to estimate the amount of H2 and thus the total mass of ISM clouds.
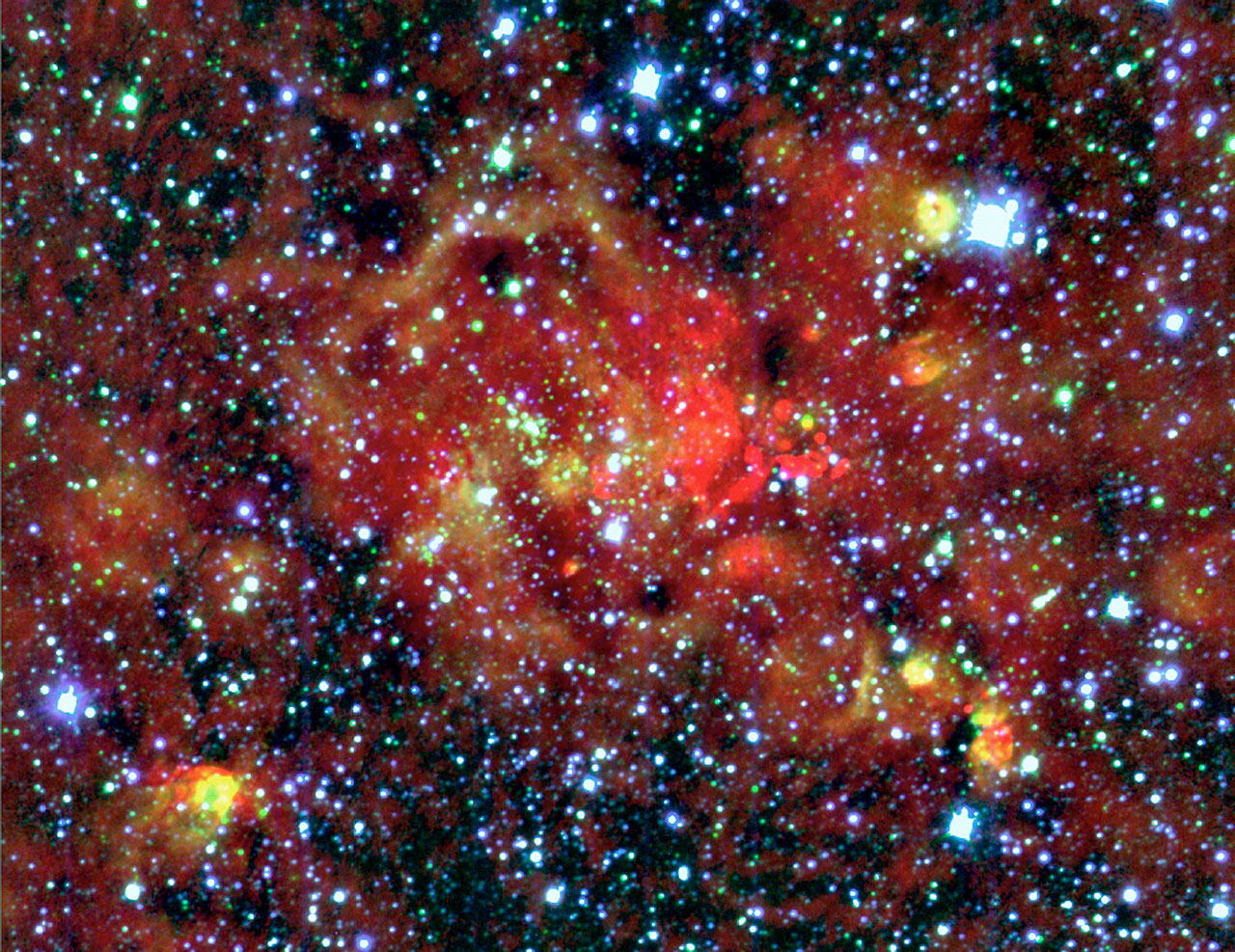
Composite near-infrared and radio-wavelength image of the Westerhout 49 (W49) star-forming region. The target of the SOFIA/GREAT observations was the upper of the two deep-red clouds of radio emission above and slightly to the right of the image center.
Sulphur-bearing molecules in diffuse molecular clouds: New results from SOFIA/GREAT and the IRAM 30 m telescope
Neufeld, D. A., et al., 2015, A&A, 577, A49
Neufeld et al. used the GREAT instrument on SOFIA to measure the amount of SH (‘mercapto’) molecules toward five star-forming regions (W31C, G29.96–0.02, G34.3+0.1, W49N and W51). [Note that Neufeld and collaborators discovered SH in the ISM using SOFIA/GREAT in 2011.]
They combined the SOFIA observations with measurements from the IRAM radio telescope in Spain of H2S, CS, SO, and CH molecules in the same regions. They also used IRAM to measure the amount of CH as a tracer / proxy for the underlying mass of hydrogen molecules (H2).
Their results indicate far more sulfur-bearing molecules than are predicted by models of “cold” ISM chemistry. This result can be confirmed by similar measurements of other star-forming regions. It indicates that “warm” chemistry and/or the effects of shock waves & turbulence are the most likely mechanism for sulfur molecule formation. This means that the main pathways for making sulfur-bearing molecules in the ISM, and probably therefore organic molecules, occur in substantially different conditions than the pathways for making water (cf. Wiesemeyer et al. 2016), indicating that creation of biogenic molecules in space is even more complicated than previously thought.
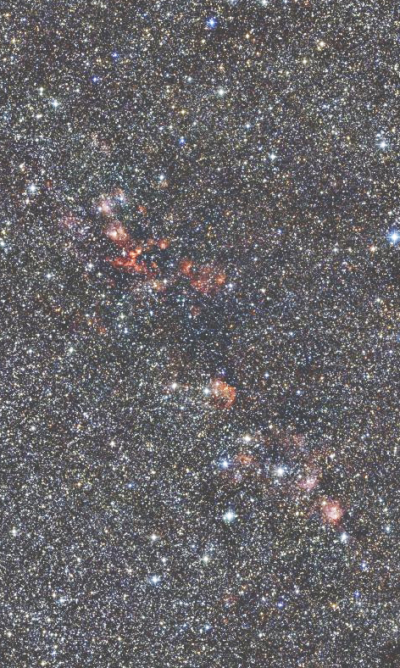
Near-infrared image of the Westerhout 59 (W51) star-forming region.
Far-infrared study of tracers of oxygen chemistry in diffuse clouds
Wiesemeyer, H., et al., 2016, A&A, 585, A76
Wisemeyer et al. investigated how water forms in the ISM by measuring the amounts of O atoms and OH molecules – from which H2O can form – in several ISM clouds. They used data from SOFIA with the GREAT spectrometer plus the APEX radio telescope in Chile. They also used data from the Herschel infrared space telescope to measure the amounts of HF and CH molecules to estimate the amount of hydrogen in the same clouds.
The ratios of abundances of H, O, and OH match laboratory experiments and models for a particlular set of H2O formation processes called “cold” chemistry rather than alternatives of “warm” chemistry or dust grain catalysis. The next step would be to confirm this result by measuring the amounts of O atoms and OH molecules in more ISM clouds. In the long run, a confirmed model of interstellar water chemistry could allow determination of how much of Earth’s water formed in the ISM even before the Solar System formed.
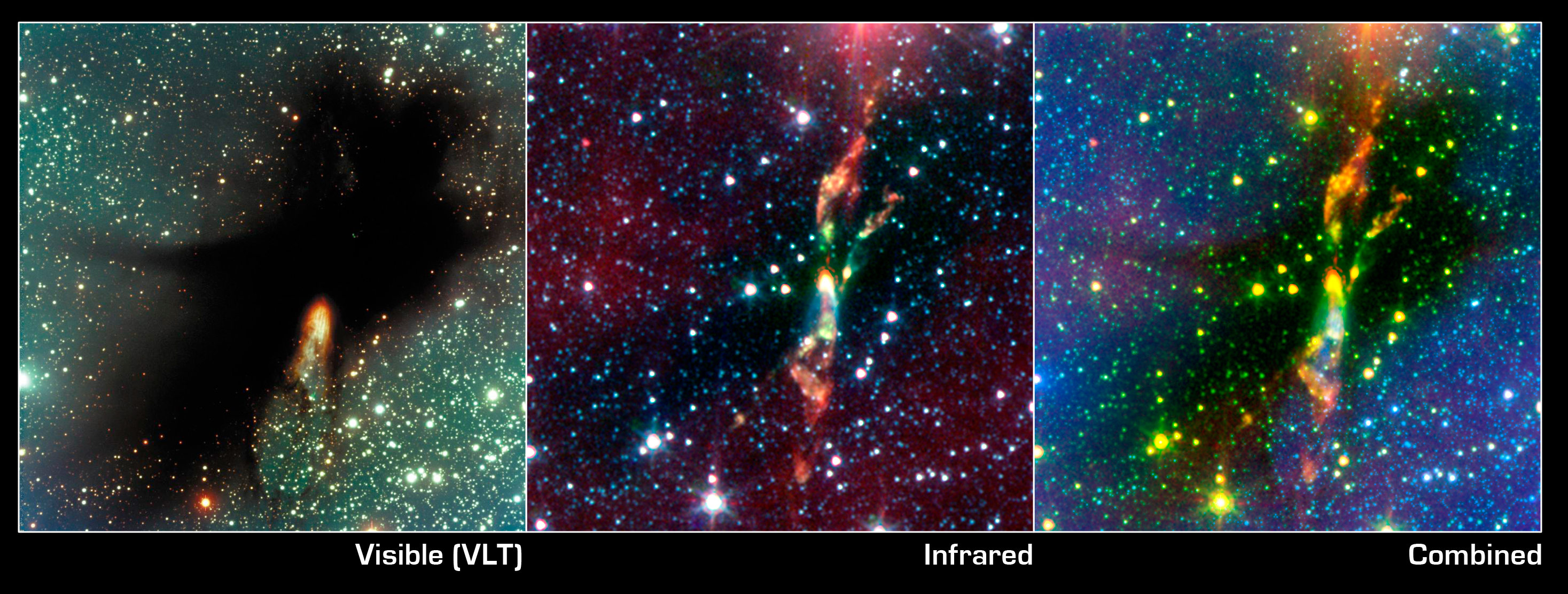
Triptych of images of the massive protostar BHR 71 and its bipolar jet; Left, visual-wavelength image from the VLT in Chile; Center, near-infrared image from the Spitzer space telescope; Right, combined visual and near-infrared.
Impacts of pure shocks in the BHR71 bipolar outflow
Gusdorf, A., et al., 2015, A&A, 575, A98
Gusdorf et al. used SOFIA and the GREAT far-infrared spectrometer to study the material within and near shock waves located where the bipolar outflow from protostar BHR71 is crashing into the surrounding ISM. They used model calculations to analyze SOFIA/GREAT observations of carbon monoxide (CO) emission plus APEX radio telescope and Spitzer space telescope measurements of CO, molecular hydrogen (H2), and silicon monoxide (SiO) to determine energy flows in the shock wave region. (The authors noted that the Spitzer measurements of H2 emission were insufficient to completely characterize what is going on in the shock regions.)
Gusdorf et al. concluded that the BHR17 bipolar outfilow has lasted for about 4000 years (a longer time than was inferred by previous studies) and has dumped a total of 4 x 10 43 ergs of energy into the ISM, equal to 300 years of the Sun’s total power output. Stirring of gas in star-forming regions by bipolar outflows from protostars is one more on the list of effects that might slow down or even prevent star-formation spreading from cloud to cloud. Further studies will be needed to determine whether this particular outflow is net enhancing or preventing more star formation in regions adjacent to BHR71.