Researchers who have never worked in the thermal infrared before, or who have only worked with thermal infrared observations from satellites, may not be familiar with the concepts of chopping and nodding, or indeed why airborne (or ground-based) thermal infrared observations must employ such an observing technique. The process requires large overheads, making the observations inefficient, so why do we do it? The main difference between thermal infrared observations from satellites and those from the SOFIA (or ground-based observatories), are that telescopes in space are 1) cryogenically cooled to very low temperatures, and 2) located above all the Earth’s atmosphere. Below it will be explained why these two factors make such a big difference in how thermal infrared observations are performed with SOFIA versus space-borne telescopes.
Let’s first look at the effects of a cryogenically cooled space telescope versus a non-cryogenically cooled telescope like the one in the SOFIA aircraft. Wien’s Law describes the relationship between the temperature of an object and the peak wavelength of the emitted radiation of that object. In the case of SOFIA, the telescope (which is passively cooled by the surrounding air) operates at about 250 K when at high altitude, which translates to a peak wavelength of emitted radiation of 11 μm. This peak wavelength of emission from the telescope just so happens to lie right within the operational range of FORCAST, which is sensitive to 5 – 40 μm radiation. Therefore, while observing with FORCAST, in addition to the radiation from the astronomical object of interest, the instrument is also detecting copious emission from the telescope itself. For comparison, the Spitzer Space Telescope operated at a cryogenically-cooled temperature of only 5.5 K. At this temperature the thermal emission from the telescope peaks at about 525 μm, and thus very little telescope background radiation is seen with Spitzer’s instruments which were sensitive from 3 to 180 μm.
Now let’s look at the effects of observing through the Earth’s atmosphere rather than above it. Even at high altitudes, the atmosphere is relatively warm, and during observations is at the same temperature as the telescope (approximately). Therefore, like the telescope, the atmosphere will emit radiation in the thermal infrared. In fact, for most typical observations, the combination of sky background emission and telescope background emission dominate the emission from the astronomical object of interest. For astronomical objects of average brightness, the FORCAST detector will observe 1 source photon for about every 100,000 background photons! Hence, ground and airborne thermal infrared observations are said to be "background-limited".
In addition to problems created by the high emission from the telescope and sky, these sources of emission are also variable. During flight, the measured atmospheric temperature is always changing slightly, due to a variety of reasons, including changes in aircraft altitude and latitude, and flying through convective atmospheric cells. Since the telescope is passively cooled by being open to the high altitude air temperature, its temperature will be variable also, with corresponding fluctuations in emission. Typically this telescope background variability is relatively long term, with measurable differences occurring on time scales of a few 10s of seconds. For the sky background emission, there is the additional effect of water vapor in the atmosphere, which is highly emissive in the thermal infrared. Therefore, any changes in the level of water vapor overburden will also affect the level of observed radiation by a thermal infrared detector. Though the water vapor overburden is much smaller at the high altitudes that SOFIA flies than even at the best ground-based observatory sites, some water vapor is still present and highly variable. Typically, the time scale of this sky background variability is relatively short, with measurable differences occurring at the rate of 1-5 Hz.
Sky background can be suppressed with a technique called chopping, in which the telescope's secondary mirror is oscillated between two positions on the sky at a frequency of several Hz (i.e. faster than the sky background rate of variability). The detector alternately views two fields on the sky; we will call these Chop1 and Chop2. At the start of an observation, Chop1 is "on-source," in other words, pointing to a field containing the object under observation, and Chop2 is "off- source", pointing to a nearby field of blank sky. By subtracting the blank sky field of Chop2 (containing sky emission) from the field of Chop 1 (containing sky emission but also the astronomical source), the sky emission is effectively removed.
However, these two chop positions of the secondary mirror give slightly different optical paths through the telescope, and therefore the observed telescope background emission is slightly different in the Chop1 and Chop2 fields and is not subtracted off in the chopping process. To remove this remaining telescope emission, the telescope is nodded, which is a physically small slew of the telescope that is performed 2-4 times per minute (i.e. faster than telescope background rate of variability). We will call the first position of the nodding process NodA, and the second NodB. The chopping configuration (i.e. the chop angle and distance) is not changed as the telescope goes from Nod A to NodB so that both nod positions sample the same two telescope optical paths in their Chop1 and Chop2 positions. This in essence creates four fields: NodAChop1, NodAChop2, NodBChop1, and NodBChop2. An example of these four fields as they would appear on the sky is given in the picture below:
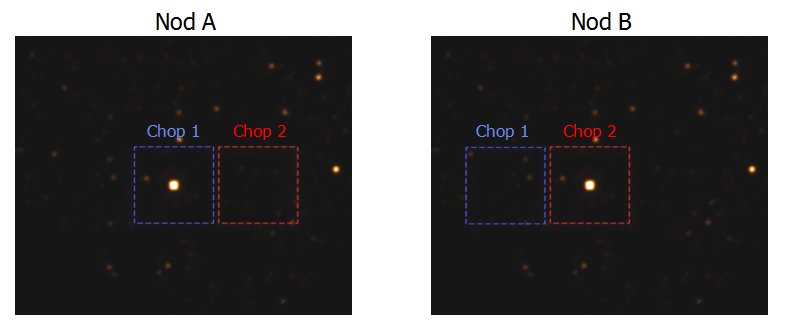
In the example pictured above, the nod is exactly equal but in the opposite direction as the chop, which would place the astronomical source at the center of NodAChop1 in the same spot on the detector when it is pointing to NodBChop2. The reference fields lie on either side of the field containing the astronomical source with no overlap (a.k.a. "off-chip chopping"). This is only one example of several ways one can chop-nod (this particular set-up we call the Nod-Match-Chop or NMC mode), however they all yield the same result: an image of an astronomical object with no sky or telescope background emission. How the data sampling sequence would appear on the sky is demonstrated in the animated image below (you may need to click the refresh button on your browser to restart the animation). Several chop cycles are performed in one nod position (at a rate of 1-5 Hz), then after about 30 seconds the telescope is nodded (i.e. when the two chop fields appear as dashed lines in the animation), and then the same number of chop cycles (using the same chop frequency, angle, and distance) are performed in the second nod position.
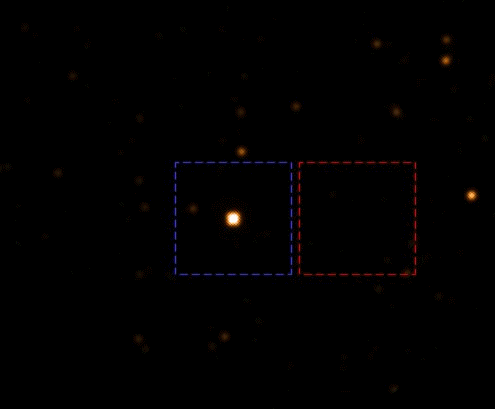
By using a double difference of these four fields, one can remove the telescope emission as well as the sky emission. Mathematically, this works out like this:
Net Signal = (NodAChop1 – NodAChop2) - (NodBChop1 – NodBChop2)
where, given the example pictured above:
- NodAChop1 contains the astronomical source emission, S, the sky background emission, BGs, and the telescope background emission through the Chop1 secondary mirror position optical path, BGt1;
- NodAChop2 contains the sky background emission, BGs, and the telescope background emission through the Chop2 secondary mirror position optical path, BGt2;
- NodBChop1 contains the sky background emission, BGs, and the telescope background emission through the Chop1 secondary mirror position optical path, BGt1;
- NodBChop2 contains the astronomical source emission, S, the sky background emission, BGs, and the telescope background emission through the Chop2 secondary mirror position optical path, BGt2.
This means therefore:
Net Signal = [(S + BGs + BGt1) – (BGs+ BGt2)] – [(BGs + BGt1) – (S + BGs + BGt2)]
All sky background terms subtract out leaving:
Net Signal = [S + BGt1 - BGt2 - BGt1 + S + BGt2]
All telescope background terms also subtract out leaving just the astronomical source emission:
Net Signal = S + S
This can also be explained pictorially with the following figure, which shows real imaging data from the mid-infrared instrument OSCIR , as it observed the galaxy NGC 253 at 10 μm from the IRTF telescope (Credit: U. Florida):
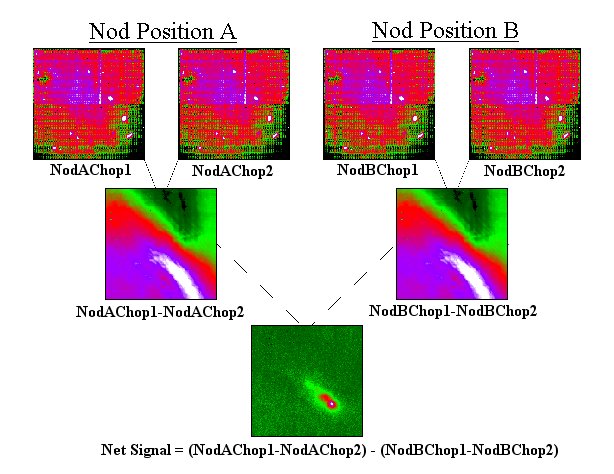
- (Top) The four raw field images as seen in the thermal infrared: NodAChop1, NodAChop2, NodBChop1, and NodBChop2. Since the sky and telescope backgrounds dominate the flux in all four images, they look like flat-fields indistinguishable from one another. In this case, each of the four images shown are coadditions of ~15,000 frames each with an exposure time of 20 msec, yielding a total integration time for each image of ~ 5 minutes. The mean signal level in each frame is ~ 2.2e11 e-.
- (Middle) The chopped-differenced images constructed from (NodAChop1-NodAChop2) and (NodBChop1-NodBChop2). In these images the sky background emission has been essentially removed, leaving the telescope background emission which has structure and exists at a level much higher than the emission from the astronomical object being imaged. The peak-valley variation is ~ 2e9 e-, only 1% of the signal of the images in the top row, but still a few orders of magnitude above the background limit of 4.7e5.
- (Bottom) The final net signal image showing NGC 253. This is produced from the differencing of the two chop-differenced images in the middle row. This has now removed the telescope background emission, finally revealing the emission from the astronomical object of interest. The signal at the peak of the galaxy's nucleus is 6.0e7 e-. The noise level is about 6.6e5 e-/pixel, in good agreement with the expected background-limited performance of 4.7e5.
There are several ways to chop and nod; some methods are more efficient than others, however. Details on the different ways SOFIA can chop and nod with FORCAST are given here , and examples similar to the one presented above with comparisons of the effective S/N of each method can be found here .
In spite of the discussion above, one might still think that nodding alone may be sufficient to subtract the background, especially in the case of imaging a nearly point-like object where the surrounding field can be used to zero the sky. Several groups have attempted this, but each have found that the background varies so irregularly over the field after just a few seconds that it cannot be modeled and subtracted to better than 1 part in 10,000. So, all groups with mid-IR imaging systems continue to chop and nod. The only exception is for high resolution spectroscopic work, where the contrast between an emission line and the background can be much higher and is therefore often done without chopping.